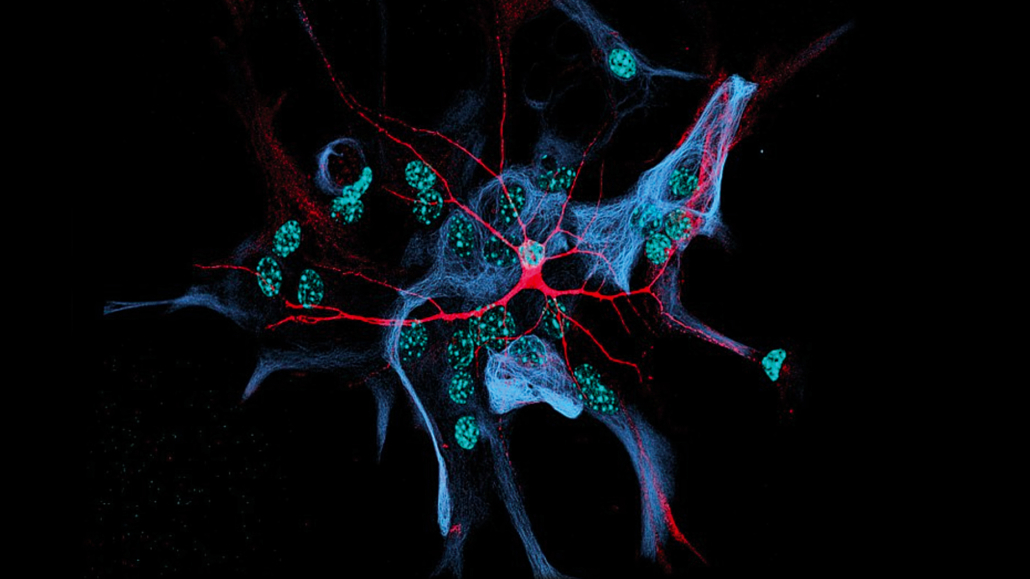
Playing God with the fates of cells
Dreams of simple, reliable and scalable processes for the much-hyped cell therapy market have generated demand for cost-effective, GMP-compliant manufacturing methods. The drug-screening market is also hungry for material that will allow the establishment of human cell disease models. A growing number of companies are now trying to coax induced pluripotent stem cells (iPSCs) into specific cells needed for clinical trials. Others are seeking the same goal through direct transdifferentiation protocols. What method will prove superior?
For stem cell researchers, a July press release from Takeda Pharmaceuticals sounded a little like science fiction. The Japanese pharma giant announced that the world’s first Car-T cell therapy produced from iPSCs had entered GMP development, and will be available for clinical testing by 2021. Takeda obtained the T-cell material for its iCARTs’ under a licence agreement with Kyoto University. There, 2012 Nobel Prize laureate Shinya Yamanaka runs a clonal master iPS cell bank that’s been funded with US$250m of Japanese government funding. The goal is to create off-the-shelf cell therapies that can be tailored to patients on demand.
In 2006/07, the Japanese researcher was the first to discover which factors can reprogramme adult mouse and human cells into iPSCs – an epigenetically native stage in which progenitor cells can proliferate indefinitely, and at least theoretically differentiate into every known type of cell. If he’s now managed to create CAR-Ts from iPSCs, Yamanaka would have overcome a major roadblock to current CAR-T and cell therapies: their high cost. Although the media has trumpeted the promise of cell therapies as the first few approvals are granted, it’s still unclear whether patient-derived treatments really offer a path forward in business terms. After all, production costs remain very high due to the extremely laborious, slow and only haltingly effective procedures used to produce enough GMP-compliant cell material (see European Biotechnology, Autumn 2010 edition). The iCART programme demonstrates the value of our T-CiRA collaboration – applying iPSC technology to develop new approaches to drug discovery and creating a bridge to transfer promising programs to Takeda to accelerate them toward clinical development and therapeutic use, Yamanaka claims. The idea behind using iPSCs for cost-effective CAR-T off-the-shelf manufacturing is to expand a single genetically engineered iPS cell clone from the iPSC master bank. That could lead to a more homogeneous allogeneic therapy than one based on immunogenic donor cells – a huge business opportunity. iCART is only one of five planned first-in-human studies in the next two years that are being fed by Yamanaka’s cell resource.
Building resources for cell supply
In the US and Europe, similar projects mirroring Yamanaka’s clonal master iPS cell bank activities are underway. The CEO of Allogene Therapeutics Inc, David Chang, called the use of iPS cells for allogeneic therapies the future of cell therapy when announcing a partnership with iPSC pioneer Notch Therapeutics Inc in early November. The cooperation aims to create allogeneic CAR-T cells from iPSCs for cancer therapy. As far as I know, we are the most advanced entity in Europe building a GMP-compliant library composed of HLA-homozygous hiPS cell banks, says Boris Greber, CSO of Rheincell Therapeutics GmbH. Like Yamanaka, the company based in Langenfeld in Germany wants to provide allogeneic cells that minimise immunosuppression requirements with implanted cells that match most HLA genotypes in Caucasian patients in the US and the EU. Our concept is based on cells derived from umbilical cord blood with HLA-homozygous genotypes. We have calculated that 20 such rare but naturally occurring genotypes would match over 60% of the Caucasian population, because one acceptor allele is sufficient for a match, says Greber. The stem cell researcher has worked for 15 years on cell reprogramming and differentiation, partly in the lab of iPSC pioneer Hans Schöler. Our goal is to further reduce complexity in these cells by genetically modifying them under GMP. That would mean we could target 90% of the world’s population with even fewer cell lines, says Greber.
iPSCs – the way forward?
There are very few estimates available on potential market sizes and revenues for standardised cell manufacturing methods. But cell manufacturer business will likely grow in lockstep with the cell therapy market, which is expected to reach US$35bn by 2023. And cell line development for industrial bioprocessing and research tools is projected to reach US$2bn by 2031. In addition to classic Yamanaka-style’ reprogramming via iPSCs, a second technological process known as transcription factor-mediated forward programming could also potentially deliver GMP-compliant, quality-controlled cells for clinical trials or drug discovery.
Human cells are incredibly difficult to source, says Mark Kotter, Founder and CEO of Bit Bio Ltd, formerly known as Elpis Biomed Ltd. The company, initially named after the spirit of hope in Greek mythology, was recently relaunched as Bit Bio Ltd. That reflects the fact that the specialist for direct iPSC reprogramming has moved beyond hope and is now ready to deliver products. It has been difficult to acquire sufficient numbers of cells from individuals. The alternative – creating cells from iPSCs – has been hampered by problems like inconsistency, as well as lengthy and complex protocols that are difficult to reproduce and scale. Seeking to overcome bottlenecks to efficient reprogramming, researchers have identified two key issues:
- transcription factors determine cellular identity
- gene silencing is the main issue holding back reprogramming,
To solve those problems, Bit Bio developed a platform for identifying cell type-relevant transcription factors using a combination of screening and machine learning. To overcome gene silencing, Bit Bio applied its Opti-ox technology. In brief, the technology places a gene switch and a transcription factor programme into two separate safe harbour sites in the genome. The switch can be flipped by a drug, so on command, Bit Bio is able to activate a chosen set of transcription factors that determine a specific cell’s developmental fate. According to Kotter, Bit Bio is already marketing iPSC-derived myocytes, neurons, and has also managed to produce oligodendrocytes. The next generation of medicine hinges on widespread access to human cells, said Kotter. That is the challenge we have set out to solve at Bit Bio. Using Opti-ox, we can produce cells in days instead of months.
A UK-based transdifferentiation specialist, Mogrify Ltd, is taking a different approach. The company relies on data of transcription factor patterns from the FANTOM5 consortium to directly convert cells without involvement of iPSCs into other cell types. But its goals and ways it seeks to address markets are the same as Bit Bio: R&D, drug discovery and cell therapy.
iPSCs: still the better alternative?
Many experts in the field, however, don’t believe transdifferentiation protocols will in the long run be able to supplant iPSC-based reprogramming and differentiation methods. A major challenge of cell programming is the choice of the method used, says Oliver Bruestle, CEO of German Life & Brain GmbH. The starting cell – whether iPSC or somatic cell – must be converted to the desired target-cell type by means of adequate differentiation triggers like transcription factors or morphogens, which change the cell’s epigenetic programme. Since different cell types have very different epigenetic signatures, a specific transcription factor combination may work for one reprogramming scenario, but fail for another. Therefore, depending on the source and target cell, a suitable programming scheme must be used or even newly developed.
According to Rheincell’s Boris Greber, classic differentiation of human iPSCs has clear advantages over transdifferentiation: You don’t need to manipulate the cells genetically, which regulatory authorities will always view as a potential safety risk, he argues. And if you don’t work with a clonal approach, he adds, you might have variation in differentiation outcomes. With an hiPSC intermediate, we have the big advantage of being able to thoroughly assess the quality of a clonal cell line, as we do at Rheincell.
In addition, of course, hiPSCs essentially don’t age under appropriate conditions, so you can easily expand them to the large numbers required for off-the-shelf cell therapeutic products. Mark Kotter is not convinced: European regulators now actively encourage gene edits, i.e. the inclusion of safety switches, and any other factors that stabilise cell types, he says, pointing to the market authorisation of CAR-T cell therapies. The possibility of one day creating off-the-shelf iPSC-based cell therapies and cell models has attracted industry attention. Proponents there take a different stance on quality requirements for cell production than academics.
For some cell types, differentiation protocols can take many weeks, says Bruestle. Reproducibility typically decreases with the length of the procedure. Here, the use of transcription factors to forward-programme iPSCs into the desired cell type can increase speed and fidelity of the process – an approach that combines the advantages of the iPSC route and transcription factor-based fate-shifting.
Hamburg-headquartered drug discovery specialist Evotec SE is pursuing iPSC-based cell models. It established a strategic alliance with Celgene Corp in 2016 to identify disease-modifying treatments for a broad range of neurodegenerative diseases. Our goal is to understand and to develop cures for human disease using patient-derived iPSC based models. With their potential to generate any human cell type, iPSCs provide a unique opportunity to develop more physiologically relevant disease models than previously available, says Sandra Lubitz, Vice-President Stem Cell Biology at Evotec. However, it is necessary to overcome technical challenges in culturing and differentiating such cells at industrial scale. In order to establish human cell models for drug screening, we have established standardised, robust and scalable protocols that deliver large amounts of quality-controlled cells.
Evotec’s iPSC platform integrates innovative technologies to define relationships between the genetic cause of a disease and resulting perturbations of specific pathways in disease, i.e. transcriptome and proteome analyses. The company also addresses limitations of iPSC-based models such as its loss of epigenetic marks during reprogramming.
As iPSC-derived neurons might represent a somewhat more immature developmental stage as compared to fully developed cells in a patient, we include studies of aging through prolonged culture or cellular stress, says Lubitz. Moreover, we also test direct reprogramming of aged adult fibroblasts into neurons, which retains the mature epigenetic signature of the cells that is otherwise lost throughout the reprogramming process. More complex cell models, such as co-culture systems and three-dimensional organoids, deepen our understanding of disease and help to select molecules for clinical phases. Our aim is to make existing protocols and techniques available on an industrial scale to advance the drug discovery process for the benefit of patients, she concludes.
Experts in the field say setbacks in the first clinical trials with iPSC-derived cells is to be expected from a technology that’s just 20 years old. Most stem cell projects are still in the discovery phase or early development. Japanese researchers have run clinical tests on cell replacement therapies in age-dependent macular degeneration (AMD) and heart failure patients, but with limited success. Australian company Cynata Therapeutics is also in Phase I trials using iPSC-derived mesenchymal stem cells to treat graft-versus-host disease. CROs and CDMOs for cell supply include Fujifilm subsidiary Cellular Dynamics International and Yamanaka’s ReproCell. Bit Bio CEO Kotter believes scalability problems will grow more apparent when cell therapies approach Phase II-III.
Taking aim at realistic targets
Oliver Bruestle – who at Life & Brain GmbH implemented a StemCellFactory’ for automated cell reprogramming – believes on the other hand that since iPSCs can be scaled very well, especially in the bioreactor, batches in the 107-108 scale could be easily produced today. That might already suffice for some biomed applications. Often, however, technical feasibility isn’t the decisive factor, but the still very high production costs, he says, particularly in high-throughput screening.
The industry is still in the midst of a steep learning curve in establishing human disease models, and big challenges remain. According to Greber, a growing number of very good protocols can coax iPSCs into a range of cell types. However, getting them to take on the roles of mature cells in a new tissue environment (niche’) is another issue. In the heart, for instance, new stem cells have to be electrically aligned with the surrounding cells. Mimicking conditions in this specific niche made iPSC-derived heart cells mature faster in culture.
There’s a lot left to do, says Bruestle. Looking at the state of development of individual technologies, iPSC-derived cell populations will initially be the focus of application, he believes. Due to the relevant complex regulations for cell therapy, this area is likely to prefer transgene-free and preferably small molecule-based differentiation methods for the time being. And they may be supplemented in the medium term by new forward-looking programming methods.
first published in European Biotechnology Winter Edition 2019