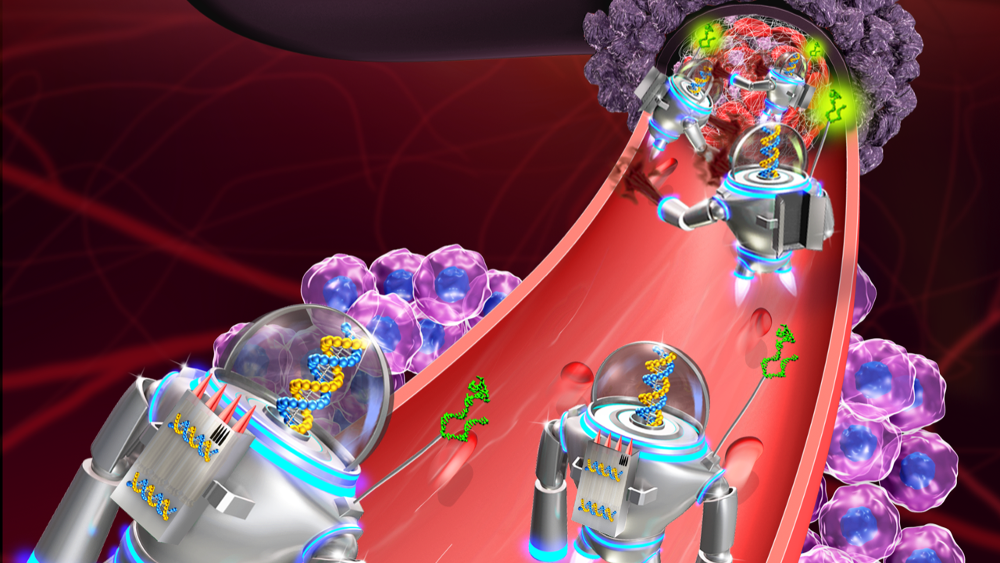
Nanobots: Fiction becoming reality
Once confined to speculative and science fiction, miniature micro- or nanobots targeting pathogens or conditions directly have now become reality in research labs worldwide. But to enter clinical trials, scientists still have to overcome two major hurdles - visualising the tiny machines inside the body, and preventing a potential immune response.
What can we really do to fight cancer? Bradley Nelson posed that question in a speech at the annual World Economic Forum in Davos 2016. And the Director of the Multi-Scale Robotics Laboratory (MSRL) at ETH Zurich is far from the only one asking that question. Scientists all over the world are feverishly searching for new ways to beat one of humankinds oldest enemies. Blunt tools like chemotherapy and radiotherapy are often ineffective, and they destroy a lot of healthy tissue in addition to the malignancy. So what if we could come up with tiny, intelligent machines – just millimeters to nanometers in size – that were programmed to kill only tumor cells? Where once only sci-fi authors dared to tread, researchers are now taking huge steps forward, designing micro and nanobots that can be used to carry and release drugs throughout the body, and specifically home in on malignant cells.
A study published in February by Arizona State University (ASU) and the Chinese National Center for Nanoscience and Technology (NCNST) showed how the partners successfully programmed DNA nanobots to shrink tumors by cutting off their blood supply (Nature Biotechnology, DOI:10.1038/nbt.4071). We have developed the first fully autonomous, DNA robotic system for a very precise drug design and targeted cancer therapy, said Hao Yan, Director of the ASU Biodesign Institutes Center for Molecular Design. Each nanobot is made from a flat, rectangular DNA origami sheet thats 90 nanometers long and 60 nanometers wide. Attached to its surface is a key blood-clotting enzyme called thrombin. The enzyme can block tumor blood flow by clotting the blood within the vessels that feed cancer growth, causing localized embolisms that lead to the death of its tissue. In mice, the researchers showed that treatment successfully blocked tumor blood supply, and generated tumor tissue damage within 24 hours – with no detectable adverse effects on healthy tissue. After attacking tumors, most of the nanorobotic systems were also cleared and degraded by the body within 24 hours. This technology is a strategy that can be used for many types of cancer, since all solid tumor-feeding blood vessels are essentially the same, says Yan.
Inspired by Hollywood
The idea of miniaturised robotic doctors taking a journey into the depths of the body to solve a health issue was first popularised by Hollywood in the 1960s. In the film Fantastic Voyage, scientists were shrunk to microscopic size along with a submarine and implanted in the body of an injured scientist to perform delicate brain surgery. During their mission, the crew faces many obstacles, including an arteriovenous fistula that forces them to detour through the heart, and they only survive when full-scale doctors in the macroworld induce temporary cardiac arrest in the patient. But finally, of course, they manage to reach the blood clot in the scientists brain and are able to successfully break it down. Filmmakers, however, have an advantage that scientists dont have. They arent restricted by the laws of physics – and what happens when things get very, very small.
When we talk about nanobots were referring to devices that exhibit at least one dimension in the nanoscale, says Dr. Salvador Pané i Vidal, Senior Research Scientist at the MSRL in Zurich. Nanobots shouldnt be confused with so-called nanocapsules or nanocontainers, which are passive systems that are already finding applications in medicine today. The term nanobots refers to active, intelligent nanomachines that would travel through the body on a certain mission – whether its the targeted transport of drugs to diseased cells, diagnosing conditions or perhaps one day even performing surgery at the microscale.
Scientists working in the field of micro and nanodevices for targeted drug delivery have spent a lot of time on the locomotion problem, trying to find good ways to make the bots move. One effective solution is based on bacteria that swim with a corkscrewing rotation of flagellar filaments. Weve understood many things. For example, how locomotion can be beneficial, how to shape these structures at micro- and nanoscales, and how to functionalise them – for example, for drug transport, says Pané i Vidal. Different researchers have worked on many of the aspects individually. For instance, we started by investigating locomotion at small scales, but now we need to focus on other aspects such as corrosion, biodegrability or multitasking. All these features have to be synergistically integrated and evaluated for each specific application. The senior research scientist recently received an ERC Consolidator grant for his project Highly Integrated Nanoscale Robots for Targeted Delivery to the Central Nervous System. Together with Bradley Nelson at the MSRL, his team designs porous structures that can load therapeutic payloads, which are then remotely controlled by magnetic fields. These fields can be used to move the structures wirelessly, or to get them to generate fields of their own. Perfecting such systems will allow the researchers to use the same source of energy for both locomotion and triggering functionalities, such as on-demand release of drugs and cell electrostimulation. What Im trying to do is to design nanorobotic structures that can navigate through the cerebrospinal fluid and repair damaged neural circuitry by electrostimulation, explains Pané i Vidal. But we have to take into account that the structure has to swim in the complex milieu of the cerebrospinal fluid, and we still dont know every kind of interaction nanobots will have to face. Follow-up tests will examine how the drug delivery systems interact in vitro with cells of the cerebrospinal fluid, as well as how they move in a pulsating flow.
Getting them out of the body
s in most Hollywood blockbusters, the characters in Fantastic Voyage have to struggle for their lives before reaching their happy end, and not everyone makes it. The mini-scientists are attacked by white blood cells, one character is killed, and their tiny sub is destroyed. In a last-ditch attempt at escape, the remaining members of the expedition swim to one of the eyes and are finally able to get out of the patients body through a tear duct. But what happens in real life to nano or microrobots placed in the body after they fulfill their appointed tasks?
Before any of the tiny devices can be used in tests involving humans, researchers in the field have to have a reliable way to remove or stop them. Even if it sends a therapy back to the starting point, researchers will have to build in safety switches that can turn everything off. But that could prove to be a very tedious and complex procedure, especially when many nanobots have been introduced.
There are different approaches to making devices biodegradable and bioabsorbable. Together with Li Zhang from the Chinese University of Hong Kong, Kostas Kostarelos from the University of Manchester for example created microbots made from spirulina algae (Science Robotics, DOI: 10.1126/scirobotics.aau0448). By dipping millions of spirulina into iron-oxide nanoparticles, Kostarelos acquired the ability to steer the devices through magnetic fields and fine-tune the rate at which the algae degrade inside the body. Our work takes advantage of some elements offered by nature, such as fluorescence, degradability and shape. But we add engineered features such as magnetisation and biological activity to come up with the proof-of-concept behind our bio-hybrid, magnetically propelled microbots, says Kostarelos.
The nano- and microbots at the MSRL Zurich are being designed to remain in the body. When it has fullfilled its task, the degradable and bioabsorbable microbot has to be able to break into pieces, be absorbed by the body and excreted via the bodys normal elimination paths. Bradley Nelson and his team are currently using biodegradable polymers that are produced through fabrication techniques like two-photon polymerisation, a kind of 3D printing technique.
Where the tire meets the road: immunoresponse & visualisation
To enter clinical trials, future microbots will have to overcome two major hurdles. First, theyll need to be biocompatible and removable or stabilisable after use. Second, researchers need to be able to see and control them while they perform their tasks inside the body. Visualisation can be seen as a key bottleneck for the further development of micro and nanorobotic devices, says Mariana Medina-Sánchez, group leader at the Institute for Integrative Nanosciences (IIN) at the Leibniz IFW Dresden. She and her team under the mentoring of Oliver Schmidt, director of the IIN-IFW Dresden, are working on speeding up sperm cells with 3D printed bots, then using them to treat cervical cancer (ACS Nano, DOI: 0.1021/acsnano.7b06398).
Radiology, ultrasound, infrared and MRI methods are too coarse, insensitive and slow to track microdevices deep within the body. Normal clinical MRI (with magnetic field strengths of up to three tesla) can resolve structures that are around 300 ?m in diameter – enough to image blood vessels. Higher magnetic fields can resolve 100 ?m, but require expensive infrastructure. A promising approach to one day visualise bots inside the body is being developed by German based iThera Medical GmbH. The team there is manipulating light, sound and electromagnetic waves to minimise the two main effects that cause images to blur: diffraction and scattering. Our optoacoustic devices work in the near infrared range, which offers the advantages of deep tissue imaging (in vivo up to 3 cm) in real time with a resolution in the µm range that is in the size of single micromotors. In addition, by the acquisition of several wavelengths and spectral unmixing, we might be able to specifically distinguish the spectral signatures of the micromotors from intrinsic tissue molecules in future in vivo studies, claims Jing Claussen, an applications specialist at iThera who is working together with the IIN and Medina-Sánchez to track and visualise the spermbots. We are conducting experiments to determine the spatial and temporal resolution of this technique. So far it looks promising compared to infrared or ultrasound imaging modalities, according to Medina-Sánchez.
Natural swimmers against cervical cancer
Sperm cells are great candidates for drug transport and delivery in the female reproductive tract. Compared to other cellular drug carriers, they have the ability to swim in viscoelastic and intricate environments, and deliver drugs into cells through membrane fusion, says Medina-Sánchez. Her spermbots are loaded with an anticancer drug (doxorubicin hydrochloride, DOX) and integrated with engineered magnetic caps that function as an artificial prostheses. Thanks to the magnetic harness, the scientists are able to now guide the biohybrid sperm cell towards an tumor spheroid cultured in vitro, and then cut it free to deliver the drug locally. Although the technology has not yet been demonstrated in vivo, Medina-Sanchez is optimistic that one day, such sperm-hybrid systems could help with assisted fertilisation and targeted drug delivery – particularly in the sperms natural environment, the female reproductive tract. The spermbots could be injected into the uterus through a catheter.
Big challenges for small bots
All scientists working in the field of nano or microbots face identical hurdles. The main challenges are the control and imaging in vivo, as the current imaging techniques lack spatial and temporal resolution. Additionally, materials and structures should be deeply analysed in the areas of undesired immunoresponse, cytotoxicity and biodegradability effects, among other factors, notes Medina-Sánchez. Microrobotics researchers, materials scientists and bioimaging and medical specialists will have to work together to solve those problems. Regulatory agencies also need to put directives in place for testing therapeutics that are based on microbots. The goal is a microbot that can sense, diagnose and act autonomously, while people monitor it and retain control in case of malfunction, said Medina-Sánchez and IIN IFW director Oliver Schmidt in their Nature commentary (DOI: 545, 406-408,2017).
Whether theyre made of DNA, polymers, microorganisms like spirulina or sperm cells, researchers have come a lot closer in the last few years to making a fantastic voyage of their own.
First published in European Biotechnology Summer Edition 2018